Read more articles from this special collection hosted by the APSF on Pulse Oximetry and the Legacy of Dr. Takuo Aoyagi. |
Oximetry: Principle, but No Theory
I am from a generation that experienced anesthesiology before the development of pulse oximeters. I was studying abroad in North America from 1973–1977, when Takuo Aoyagi, PhD, envisioned the principle of pulse oximetry. This was just about the time when Minolta began selling a finger-type device, and I knew nothing of Aoyagi’s existence or the idea of pulse oximeters. At that time, it was hard to get up-to-date information. International telephone calls cost 8,000 yen for three minutes (the equivalent of 50,000 yen or US $500 now). Japan was just starting to shed the image of Made in Japan = cheap and poorly made. The Hewlett Packard 8-wavelength ear oximeter was already in use at a research laboratory. Although it appeared accurate, it seemed to be cumbersome for clinical use.
It wasn’t until six years after returning to Japan that I met Aoyagi at a Japanese Subcommittee of the International Organization for Standardization. We attempted, unsuccessfully, to establish a standardized calibration method. In the 36 years since, I have had the privilege of learning from him, and having lived through the same generation as a clinician and a developer, I feel a responsibility to report how his great invention was born and how it grew. Therefore, I hope to use this occasion to enable people worldwide to learn how pulse oximetry, conceived in Japan, has developed and the issues left to still be resolved.
Pulse oximeters can be used on all people no matter their color, race, age, body shape, place of measurement, or type of device. By merely turning on a switch, a clear number from 0-100% is displayed and in healthy people a number that “seems right” shows up. However, according to Takuo Aoyagi, the basis for the numbers displayed just happens to make them look right. It is important to not overlook the precision and reliability of the measurement parameters, and also to understand the physiological and medical issues involved in order to correctly interpret the number displayed.
Pulse oximeters measure oxygenation, not respiration, but ordinary people and even some medical professionals tend to overlook this.1 It is a percutaneous measurement subject to various factors, but is highly reliable when there is no body movement and in patients with a good pulse. In cases of extremely low measurements, sometimes it is better to believe the numbers than the patient’s clinical presentation.2 As has been seen in the COVID-19 pandemic, patients may present with silent hypoxia3,4 without symptoms.5 Takuo Aoyagi was concerned about the lack of understanding of pulse oximeter measurements, even before the devices became popular with the public. This concern guided his research on establishing a theory of pulse oximetry in his later years. While skin color may not be a problem in Japan where there is little diversity, it is possible that other such reports will come out from other areas of the world.6
Pulse Oximetry: Two Beginnings
The invention of pulse oximetry started in Japan and is now used in both medicine and by ordinary people around the world. Surprisingly enough, two patents were filed at almost the same time in 1974. Takuo Aoyagi, on behalf of Nihon Kohden (patent filed March 29, 1974), and Akio Yamanishi, on behalf of Minolta (patent filed April 24, 1974), came upon this idea independently of one another.7,8 Aoyagi’s device, which came first, used a dye densitometer on the earlobe to measure cardiac output. He came upon his idea during an experiment to eliminate superimposed pulsation noise. His light source was an incandescent light bulb and his point of measurement was the earlobe, both of which made it difficult to develop a practical device and the project ended. Chances are that it was not pursued because the invention was a side product and did not align with the company’s main project.
Aoyagi reported on his invention to his supervisor, and it just happened that a physician the supervisor was visiting heard about it, and work on a prototype was started. They were less interested in the significance of oxygen saturation and were mainly looking at new methods of measurement. Aoyagi reported that once the paper was published, there was no more mention of turning it into a clinical device. However, Aoyagi continued his research into establishing a theory of measurement through the years, and after a break of about 10 years, Nihon Kohden renewed its development. They allowed Takuo Aoyagi to pursue his research until the end and he fulfilled their expectations. Takuo Aoyagi presented his invention, the pulse oximeter, to Japanese anesthesiologists first in 1989 at The Japan Society for Clinical Anesthesia Academic Meeting in Tokyo, Japan. However, it wasn’t until 2002, when the Japanese Society of Anesthesiologists gave Aoyagi an award for his contribution to society, that his name and Nihon Kohden’s pulse oximeter became familiar to anesthesiologists in Japan.9,10
On another front, Akio Yamanishi’s group was taking advantage of the new LED technology to develop fingertip plethysmography, and the development of a pulse oximeter was one of their primary projects. They succeeded in developing the world’s first fingertip pulse oximeter. Ikuto Yoshiya (anesthesia professor at Osaka University at the time) and Yasuhiro Shimada (assistant professor at the same university) were involved, but their contributions were limited to improving precision through analysis.11 Minolta started selling their device (OXIMET 1471) through Mochida Pharmaceuticals in June 1977, but rather than using LED as a light source, they used a combination of tungsten and fiberoptic cable, so although the device was usable, it was difficult to operate. It is possible that the red spectrum in LEDs at the time was not sufficient.
Clinical Significance Unrecognized in Japan
The OXIMET 1471 pulse oximeter that went on the market in 1977 seems to have been reviewed by several university academic anesthesiologists in Japan.12 However, while the device was judged to be useful as a research measurement device, it did not take off as a clinical one. Only 200 devices in total were sold. Kunio Suwa (associate professor of Anesthesiology at Tokyo University) tried it out in 1992 on his own volition,11-13 but unfortunately even then, as now, bureacracy existed in Japan’s medical device industry, slowing the development of innovations.
The first scientific meeting on pulse oximetry took place in Chartridge, outside London, in 1985, and the first international neonatal and pediatric meeting on pulse oximetry in Japan was held in Hakone, outside Tokyo, in 1987 (Figure 1).
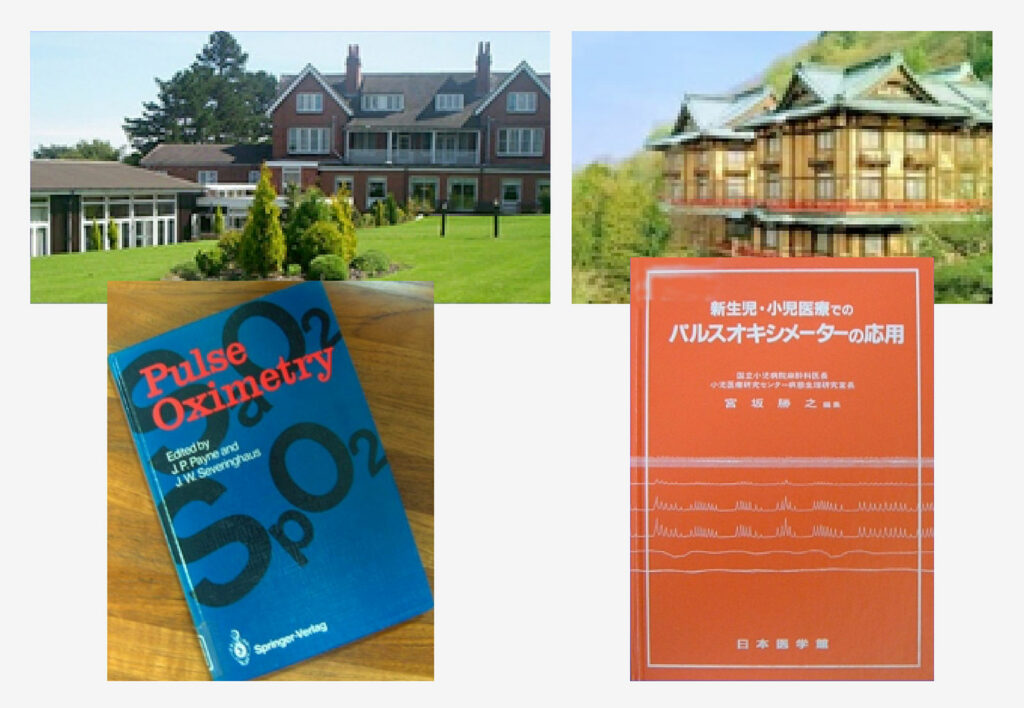
Figure 1: Top left: International conference at Chartridge Seminar House held May 1985 at a suburb of London with 50 participants—Definition of SpO2, discussion of how to think about oxygen saturation. Bottom left: Published proceedings of the Chartridge Conference. Top right: May 1987, Hakone Fujiya Hotel in Kanagawa Prefecture—International conference on neonates and pediatric patients with about 20 participants—From TcPO2 to SpO2. Bottom right: Published proceedings of the Hakone Conference on Neonatal and Pediatric Applications.
Research Measurement Devices and Clinical Monitoring Devices
Oxygenation values arbitrarily showed up from around 90% to 120% soon after attaching a probe to the patients finger in the early version of Minolta’s OXIMET-1471.12,13 The device had a calibration knob that adjusted digital number values making it possible to make appropriate adjustments at the bedside. You could set blood gas values to match the patient’s oxygenation at the start of measurement, so it would display correct values thereafter. The developers would say that if the display shows 100%, then you can believe that it really is 100%. The display also showed very specific values to one decimal point at each heartbeat (say 95.6%). In actual use, however, after calibration of the device to the patient’s blood gas levels, it was not unusual to see numbers from 100% or much more, baffling clinicians. Still, with further improvement, it was a device that had great possibilities as a monitor.
From Anesthesia to Critical Care In Japan:
The Japanese Society of Anesthesiologists created their first safety guidelines (Monitoring Guidelines for Anesthesia Safety) and recommended use of pulse oximeters during anesthesia. This was seven years after ASA released their first Monitoring Guidelines for Anesthesia in 1986 in the US.14 Half of the physicians who engaged in anesthesia did not have access to even one pulse oximeter in their institutions. Domestic competition was practically non-existent. Interest in pulse oximeters grew rapidly in the field of anesthesia, but when their use expanded from during anesthesia when patients didn’t move to the recovery room, ICU and general wards, a big problem arose in how to deal with false alarms from body movement. When venous waves are superimposed on pulse waves, the convenient assumption of pulse oximeters that all pulsation is arterial pulsation no longer holds. In efforts to decrease false alarms, many strategies were tried such as temporarily freezing alarm information, prolonging the moving average time of the data, and extraction of the arterial waveform during synchronization with electrocardiograms, but none of these served as a fundamental solution (Figure 2).
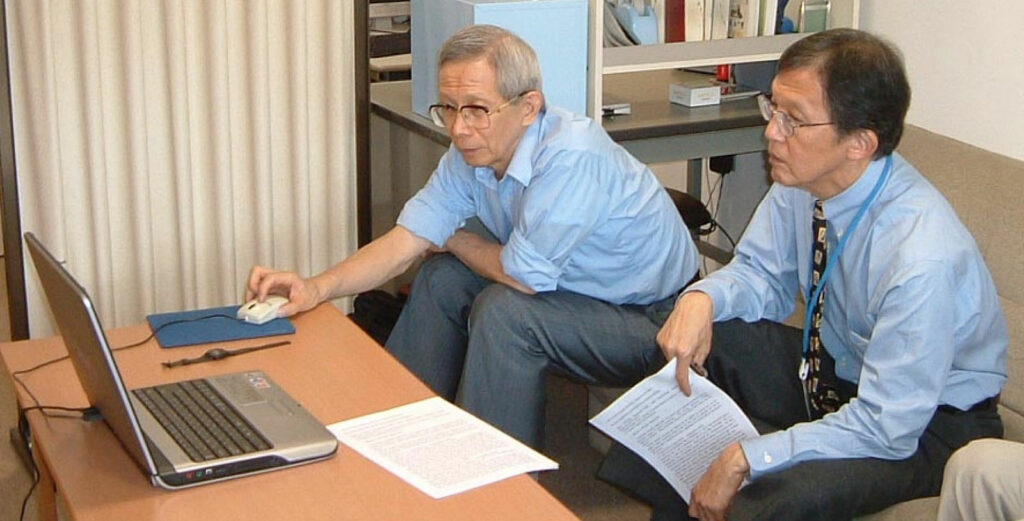
Figure 2: Dr. Aoyagi showing his work on motion artifact to Dr. Byron Aoki, from the University of Hawaii (at the author’s office when he was the head of the ICU at the National Center for Child Health and Development in Tokyo in 2002).
Emergence of A Solution to False Alarms Due to Motion
Yasuyuki Suzuki and I were studying the reliability of and problem of false alarms in respiratory monitors in pediatric ICUs at the National Children’s Hospital in Tokyo and respiratory therapy in home care pediatric patients.15 We had also introduced a project called the “Sound of Silence” to address the problem of alarm fatigue in pediatric anesthesia and pediatric ICUs such that all alarms were silenced within 3 times of beeping. Thus, we were able to obtain many hours of raw data and video recordings from pulse oximeters and patients. It was not a comparative study and it was not published, but this data on Japanese patients in pediatric ICUs helped strengthen strategies to deal with body movement, and thus low perfusion in adults.16,17
Pulse Oximetry: Problems with Multi-wavelengths and Precision
The theory of multi-wavelengths (5 wavelengths) was proposed in 200818 and was established by Aoyagi in 2015, but no product had been made, due to prolonged verification activities. In 2020, the topic of the clinical significance of measurement differences due to racial differences (skin color)6 came up, but there was little basis for discussion because there was no theory and no way to compare numbers using a standardized calibration. However, we cannot neglect differences in skin color, race, adults, infants, body shape, and place of measurement of the device. It is impossible to standardize calibration using actual measurements on human beings who can’t be standardized (no more than calibration can be standardized), between manufacturers and devices, different probes, etc. The road laid down for us by Aoyagi is of great importance to break the deadlock of the acceptance of differences of 1–2% especially in the low SpO2 range and to establish a fundamental theory of pulse oximetry.
Research on Multi-wavelengths
An in vitro calibration method19 for ISO was never established, but this was the same as saying the theory had not been established. The most recent ISO standard ended up mandating empirical calibration using blood sampling in healthy adults who are exposed to a non-physiological level of hypoxia. Thus, the accuracy of currently available pulse oximeters ignores such factors as race, age (adult or child), or individual devices. Hironami Kubota questions whether regular household devices really need to go through such a complicated calibration process. It is a very complicated issue.
Takuo Aoyagi started working on a complete theory and after verification with experiments with multi-wavelength simulation models that took into account light scattering and pulsation, and also the effect of surrounding tissue, he presented his work at the Innovations and Applications of Monitoring Perfusion, Oxygenation and Ventilation (IAMPOV) meeting in 2015 in Tokyo, Japan (Figure 3).20 The main reason for his studies using multi-wavelengths was to improve precision. But since he wasn’t looking at such factors as abnormal hemoglobin, it is possible his research was not considered important enough to result in product development.
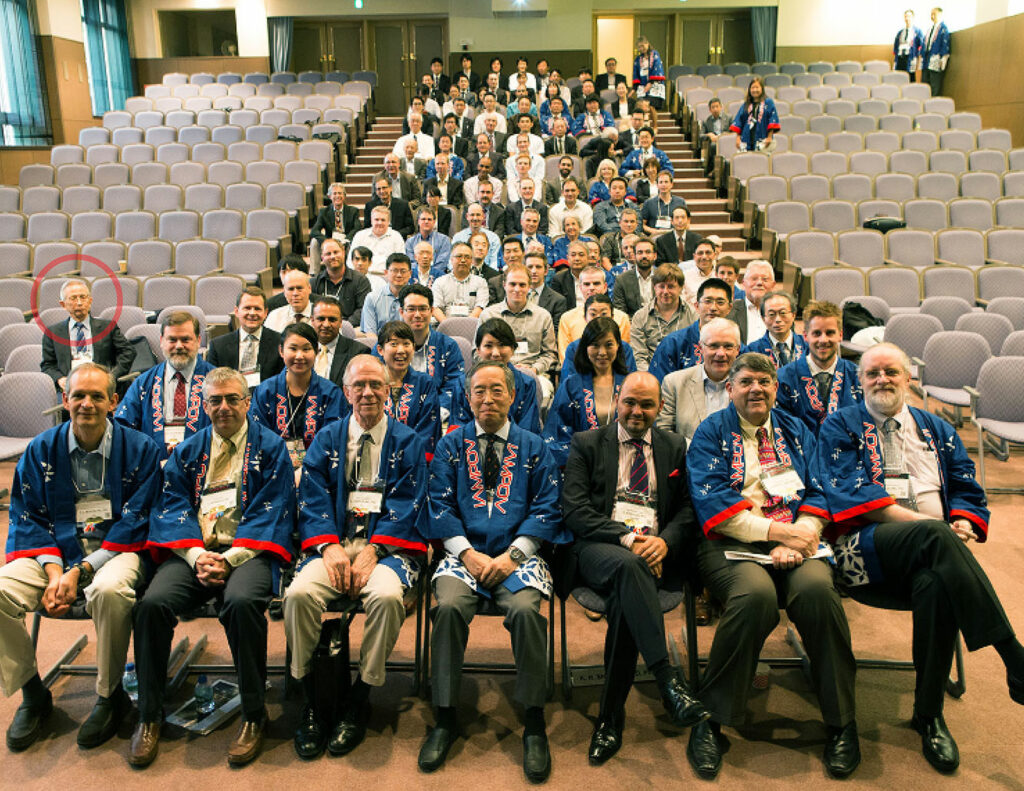
Figure 3: 2015 Tokyo IAMPOV Symposium (Last day, Auditorium at St. Luke’s International University)—An international symposium on patient monitoring devices and technology related to circulation, oxygenation, and respiration. Left side in red circle: Takuo Aoyagi. Central area, front row from left to right: P. Bickler (UCSF), S. Weininger (FDA), S. Barker (Masimo) K. Miyasaka (St. Luke’s), P. Kyriacou (U. London), B. Kopotic (Edwards), K. Shelley (Yale).
The Spread of Pulse Oximeters in Society and the Issues Involved
Aoyagi feared that without a theory, the pulse oximeter number displayed might take on a life of its own, especially with its widespread use. Under the shadow of the great usefulness of the device for COVID-19, it is a concern that pulse oximeters are being used not just for operating room patients, but everyone and without a proper understanding of what the number means. Pulse oximeters cause little harm as electronic devices, but if the numbers are misinterpreted, critical harm can result. The current regulatory system to protect users from suffering this kind of harm is inadequate.
Clinicians should help inform people of possible dangers from these devices and educate them on how to interpret the number displayed. In the current state of affairs, where the appropriate use of pulse oximeters is not guaranteed, people won’t even be able to tell if a device is poorly made as long as the number looks right. Even if there was a dangerous condition, no one would notice a problem as long as the number is within the “normal” range.
While it is necessary to educate users about correctly understanding the number, the regulations demanding proper education of the general public in Japan are vague. The manuals included in the devices say “seek a doctor’s opinion if there is a problem,” but this warning is of no use to the lay public because there is no way for them to know if there is a problem or not. Thus, the user is left believing in the device without adequate understanding, and no one including the company or government has responsibility for misuse of the device.
While the authorities in charge may be interested in the safety of electronic products, they may not be as interested in how the numbers displayed are interpreted or in the safety of the medical device. There are very few cases where clinicians are involved in product inspection. Our mission is to educate the public whenever we have a chance and provide them with the knowledge they need to evaluate products where medical quality and non-medical quality products are combined.
Conclusion
The lives of so many people have been saved and many more will be saved in the future, due to Takuo Aoyagi’s invention.
Takuo Aoyagi presented his principle of pulse oximeters for the first time in 1974. The session was chaired by Tatsuo Togawa (professor of medical engineering at the Tokyo Medical and Dental University), a prominent scientist in the field. Togawa stated in 2011 that pulse oximeters have developed much more than could be imagined even then from Aoyagi’s presentation.21 The possibilities for pulse oximetry using multi-wavelengths are many, including the establishment of a standard method of calibration, improvement in the precision of measurements during low perfusion or body movement, and by including the measurement of other substances or metabolic situations. It may even be possible for it to act like pulse spectrophotometry, by expanding measurements beyond oxygenation such as blood sugar levels, which are now measured invasively.22 This may not be as easy as it sounds to a clinician, but placing our hopes with the scientists following Takuo Aoyagi, I would like to express my gratitude for the great contributions made by him in this field.
Katsuyuki Miyasaka, MD, PhD, is executive advisor to the Dean, Wayo Women’s University, professor emeritus, St. Luke’s International University, Tokyo, Japan.
The author has no conflicts of interest.
References
- Fu ES, Downs JB, Schweiger JW, et al. Supplemental oxygen impairs detection of hypoventilation by pulse oximetry. Chest. 2004; 126:1552-8.
- Death of a male patient under observation at home. Local governor apologized. (In Japanese). https://www3.nhk.or.jp/news/html/20210109/k10012805851000.html. Accessed on Jan 9, 2021.
- Wilkerson RG, Adler JD, Shah NG, et al. Silent hypoxia: a harbinger of clinical deterioration in patients with COVID-19. Am J Emerg Med. 2020;38:2243.e5–2243.e6.
- Jouffroy R, Jost D, Prunet B: Prehospital pulse oximetry: a red flag for early detection of silent hypoxemia in COVID-19 patients. Crit Care. 2020;24:313. doi: 10.1186/s13054-020-03036-9.
- Miyasaka K: Possibility of silent hypoxia without dyspnea: Use of pulse oximeters. Yomiuri Newspaper. May 21, 2020 (In Japanese)
- Sjoding MW, Dickson RP, Iwashyna TJ, et al. Racial bias in pulse oximetry measurement. N Engl J Med. 2020; 383:2477–2478.
- Aoyagi T, Kishi M, Yamaguchi K, et al. Improving ear oximeters. JSMBE. 1974;13:90–91 (In Japanese).
- Miyasaka K. Anesthesiology and dawn of the pulse oximeter. Masui. 2018;67:S245–251 (In Japanese).
- Severinghaus JW, Honda Y. History of blood gas analysis. VII. Pulse oximetry. J Clin Monit. 1987;135–138
- Severinghaus JW: Takuo Aoyagi. Discovery of pulse oximetry. Anesth Analg. 2007; 105(6 Suppl):S1-4
- Yoshiya I, Shimada Y, Tanaka K, et al. New noninvasive oxygen saturation measurement (OXIMET): Its measurement principle and performance. ICU and CCU. 1978;2:455–460 (In Japanese).
- Suzukawa M, Fujisawa M, Matsushita F, et al. Our experience with a fingertip wave type oximeter. Masui. 1978; 27:600–606 (In Japanese).
- Suwa K (ed). Pulse Oximeters. Igakutosho Shuppan, Tokyo 1992 (In Japanese).
- Japanese Society of Anesthesiologists English Website, Standards & Guidelines, https://anesth.or.jp/users/english/news/detail/605bee53-44c0-4042-9fb0-113b9dcdd4c6#login-form-top. Accessed July 10, 2021.
- Miyasaka K, Kondo Y, Suzuki Y, et al. Toward better home respiratory monitoring: a comparison of impedance and inductance pneumography. Acta Paediatr Jpn. 1994; 36:307–310.
- Miyasaka KW, Suzuki Y, Miyasaka K. Unexpectedly severe hypoxia during sprint swimming. Anesth. 2002;16:90–91.
- Barker SJ. “Motion-resistant” pulse oximetry: a comparison of new and old models. Anesth Analg. 2002;95:967–972.
- Aoyagi T, Fuse M, Kobayashi N, et al: Multiwavelength pulse oximetry: theory for the Future. Anesth Analg. 2008;105:S53–S58.
- International Standard. ISO 80601-2-61:2017. Medical electrical equipment—Part 2–61: particular requirements for basic safety and essential performance of pulse oximeter equipment. 2017.
- Aoyagi T, Fuse M, Ueda Y, et al. Improving precision of pulse oximetry. The 4th International Symposium on Innovations and Applications of Monitoring Perfusion, Oxygenation and Ventilation (IAMPOV) 2015, Tokyo. https://iampov.org/2015-symposium/
- Togawa T. Father of the pulse oximeter. JSMBE. 2011;49:310–312 (In Japanese).
- Aoyagi T, Miyasaka K. The theory and applications of pulse spectrophotometry. Anesth Analg. 2002;94:S93–S95.
Read more articles from this special collection hosted by the APSF on Pulse Oximetry and the Legacy of Dr. Takuo Aoyagi. |